Wi-Fi 6:ファクト ファイル
Introduction to Wi-Fi
While many of us lived without it, we can’t imagine doing so today. It’s so intertwined in our daily activities, we don’t think about it anymore. Virtually every service we interact with needs Wi-Fi to work.
One of the biggest success stories of modern technology, Wi-Fi has fundamentally changed how we live, work and play. From transforming education and extending health care providers’ capabilities to pushing the boundaries for new technologies, industries and careers, it puts the world at our fingertips.
Wi-Fi will continue to revolutionize communications in ways we can’t yet imagine. It’s estimated that Wi-Fi will have a global economic value of $4.9 trillion in 2025, up from $3.3 trillion in 2021.1
オフラインで読みますか?
後でお読みいただけるよう、この記事の PDF 版をダウンロードしてください。
最新情報を常に入手
エンタープライズソースを購読すると、新しい記事が投稿されたときに最新情報を受け取ることができます。
Wi-Fi2 is a wireless networking technology that allows computers and other devices to communicate over a wireless signal. It describes network components that are based on one of the 802.11 standards developed by the IEEE and adopted by the Wi-Fi Alliance.
Nearly all modern computers have built-in Wi-Fi chips that allow users to find and connect to wireless access points. Most mobile devices, video game systems, and other standalone devices also support Wi-Fi, enabling them to connect to wireless networks as well. When a device establishes a Wi-Fi connection with a router, it can communicate with the router and other devices on the network. However, the router must be connected to the internet (via a DSL or cable modem) in order to provide internet access to connected devices.
セルラーと Wi-Fi は数十年にわたって共存しており、現在に至ってもどちらも他方に取って代わるものではありません。On the contrary, both have flourished, and it’s widely accepted that the need for multiple wireless technologies will continue. While Wi-Fi and cellular technologies are similar, they largely support different use cases. 多くの場合、これは代替品ではなく補完的なものとなっています。
セルラー
セルラーは認可されたスペクトルで動作し、モバイルネットワークオペレータ (MNO) の形でスペクトル所有者の存在を暗示します。For the subscriber, the advantage is that connection to the network is automatic, universal and pervasive. Cellular technology also has greater range to cover large spaces, and it’s inherently mobile, meaning users’ sessions are maintained even as they move between serving radios. Mobility is one of the features that makes cellular suitable for voice calling, since voice call user experience is disrupted by session interruptions, whereas many data experiences (e.g., email) aren’t. Cellular is also the technology on which emergency services organizations (fire, police, ambulances) have generally standardized their communications.
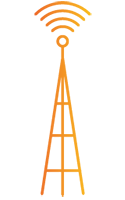
Wi-Fi
In contrast, Wi-Fi operates in the unlicensed spectrum, enabling a private enterprise or homeowner to create a network without relying on a commercial service provider. As such, it’s the default access to the enterprise or home network. It’s valued for its ability of self-deployment and absence of subscription cost. Wi-Fi テクノロジーは、アンメット高速接続を提供し、MNO 以外のエンティティによるユーザーデータ収集を可能にします。Note, however, that access to Wi-Fi isn’t automatic for everyone—only for regular authorized users. 新規または訪問したユーザー(許可されている場合)は、ログインする必要があります。
Find out more about RUCKUS®, CommScope’s wireless enterprise networks solutions
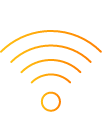
Wi-Fi was invented in 19973 and became available for consumers that year, but its origins go back much further.
Actress Hedy Lamarr and composer George Antheil developed and patented a frequency-hopping system for torpedoes during World War II4. They were unsuccessful in selling the technology to the U.S. Navy. This technology is a key component of today’s wireless data systems.
Fast forward to 1971. The University of Hawaii connected seven campuses on four islands using wireless technology that sends data as packets5. ALOHAnet and the ALOHA protocol were early forerunners to Ethernet and, later, the radio frequency 802.11 standards that are used for broadcasting a Wi-Fi signal.
It’s Vic Hayes, however, who has been called the “Father of Wi-Fi” because he developed the 802.11 standards that would make Wi-Fi feasible in 1997.
As of early 2020, the latest version of Wi-Fi was called Wi-Fi 6, a consumer-friendly rebranding of the technical format, 802.11ax. Earlier versions of Wi-Fi, such as 802.11ac and 802.11n, are now known respectively as Wi-Fi 5 and Wi-Fi 46.
The 802.11 standards address:
- How far wireless signals reach (indirectly, since it is related to power levels)
- How much data the signal can convey
This chart shows the standards name and new Wi-Fi naming conventions:
*The Wi-Fi Alliance only officially named Wi-Fi 4, Wi-Fi 5 and Wi-Fi 6. Wi-Fi 1-3 is the assumed naming convention.
As we’ve seen, a specific unlicensed spectrum band is allocated to Wi-Fi systems. Three frequency bands are currently available: 2.4 GHz (Wi-Fi 1*, 3*, 4 and 6), 5 GHz (Wi-Fi 2*, 4, 5 and 6) and 6 GHz (Wi-Fi 6E). Due to varying regulations, a general rule of thumb can be thought of as the lower the frequency, the longer the reach and the lower the maximum speed (the transmit power for each channel plays a role too). If you want more Wi-Fi coverage, you can use the 2.4 GHz band. If you want faster speeds, you can use the 5 GHz or 6 GHz band.
Within these Wi-Fi frequency bands are smaller bands, referred to as Wi-Fi channels. Channels are the means by which many devices can operate on the same frequency band in the same vicinity. Depending on the country, the 2.4 GHz band has anywhere from 11 to 14 channels, and the 5 GHz band has between 17 and 25 channels7.
Think of Wi-Fi channels as lanes in a highway, though they may have different widths:
- Pre-defined frequency ranges within a band (2.4, 5 and 6 GHz)
- Standard sizes: 20, 40, 80 and 160 MHz
- In practice, channels overlap (5 MHz spacing) in the 2.4 GHz band, so there are less of non-overlapping channels available for an access point (AP) to choose from
Just as highway lanes do, Wi-Fi channels can get congested, and managing the right channel to use can significantly improve the network performance (more about that in Part II of this article).
5 GHz Channelization
Example of channel utilization
Today, nearly every device is capable of being Wi-Fi enabled. More than 13 billion Wi-Fi devices are installed globally, and that number is increasing every day.
Wi-Fi by the numbers
Consumers were connected to an average of 6.5 devices per person in 2017.8 And it’s not slowing down. It’s expected to grow to 15 connected devices per person by 2030.9
While some of these devices will use 5G cellular technology, industry experts believe Wi-Fi will coexist with 5G10 and be a key part of many 5G use cases. More in-use devices means more demand on networks. This is where Wi-Fi 6 comes into play.
Audio and video networks
Many networks use real-time transport protocol (RTP) to deliver audio and video services.
Voice traffic has two network requirements:
- Voice traffic is sent with no expectations of receiving an acknowledgement response from the receiving client.
- Voice traffic is small in terms of data consumption. Based on the compressor/decompressor used in devices, the payload is about 64 kilobytes—and about 264 kilobytes with the addition of management headers.
Voice traffic places very little demand on network bandwidth. When latency is an issue, however, dropped calls and interference may occur.
Video conferencing applications have the same requirements as voice traffic, with the added demand of audio. Wi-Fi 6 addresses latency and jitter for both audio and video—improving application performance.
Automation
Much like voice and video, automation traffic is small in size but sensitive to latency. Networks often add automation services without consideration of whether they can support these services. As with audio and video, automation traffic doesn’t require significant bandwidth, but it is sensitive to latency.
Application solutions with Wi-Fi 6
Wi-Fi 6 uses orthogonal frequency division multiple access (OFDMA) technology to allow multiple network users to be served at the same time and with varying bandwidth needs. The more devices, the more efficient the network becomes—all while increasing speed and capacity.
モノのインターネット
The internet of things (IoT) brings together all the devices, appliances and sensors that are connected to the internet. IoT is characterized by low-bandwidth applications, low throughput and fewer latency issues.
More clients sending smaller payloads less often feeds directly into the concept of OFDMA. Being able to adapt the number of resource units on the fly could easily solve network congestion obstacles by accommodating many users simultaneously and allocating bandwidth optimally.
Wi-Fi 6 supports high-speed, low-latency connectivity and has a theoretical maximum speed of 9.6 gigabits per second11. That’s almost 2.6 times more than Wi-Fi 5.
As Wi-Fi 6 expands and becomes the new standard for networks, businesses will start migrating their infrastructure to Wi-Fi 6. This will be increasingly important in high-density environments, such as stadiums, convention centers and transportation hubs.
The new technology maintains backward compatibility for older devices while increasing capacity and security, ramping up data rates, reducing network congestion and improving battery life for compatible devices.
Wi-Fi 6 APs deployed in dense device environments will likely be required to support higher service-level agreements to more concurrently connected users and devices—with more diverse usage profiles.
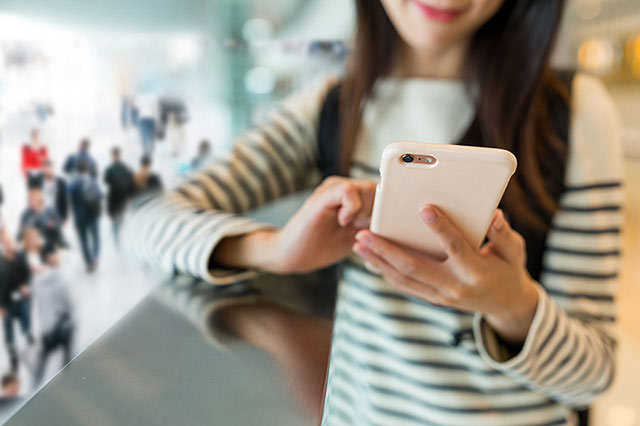
Three key technological updates contribute to Wi-Fi 6’s improved performance over Wi-Fi 5:
- Multiuser, multiple input, multiple output (MU-MIMO): Allows a Wi-Fi AP to communicate with multiple devices simultaneously, improving the overall Wi-Fi experience. MU-MIMO can significantly improve throughput in high-density networks—even in those that use bandwidth-intensive services.
- Orthogonal frequency division multiple access (OFDMA): Divides a Wi-Fi channel into smaller frequency allocations known as resource units. This allows an AP to communicate with multiple clients by assigning them to specific resource units.
- 1024-quadrature amplitude modulation (QAM): Enables a 25 percent data rate increase in Wi-Fi 6 APs and devices. By varying the phase and amplitude of radio waves, the technology improves spectral efficiency by incorporating more data into each transmission.
While Wi-Fi 6 improves speed significantly, thanks to more radio chains and spatial streams, most devices won’t approach 9.6 gigabits per second. For mobile devices, the power and antennas needed to achieve the maximum rate are prohibitive due to battery and physical space restrictions. In addition, most mobile devices can’t even use the amount of data that comes from a multigigabit connection speed.
Imagine a four-lane highway that could be expanded to eight lanes and accommodate large and fully loaded trucks. Picture the same highway with the trucks only 20 percent full. In this scenario, the possible efficiency and capacity of the network is wasted because the trucks are crowding the road without full capacity. Each truck can only carry products (or data) for one customer (one device).
The vehicles (devices) using the highway network is an inefficient system. The solution? Instead of having one truck per customer, trucking companies will have one truck pick up multiple packages from multiple customers, until the truck is full, before sending it out on the road. This allows more products (data) on the road.
Resource utilization
Combining multiple devices into a single resource wasn’t possible with earlier versions of Wi-Fi. Only one device could transmit at a time—whether it had a full payload (full truck) or the average payload (only 20 percent full). Wi-Fi 6 changes that.
Wi-Fi 6 APs with Wi-Fi 5 clients
It’s difficult to quantify the exact improvements networks will realize with Wi-Fi 6. CommScope testing has shown, however, that replacing a Wi-Fi 5 AP with a Wi-Fi 6 AP increases network speed up to 20 percent—even in an environment with all Wi-Fi 5 clients. This percentage will vary depending on differences in clients and applications used.
Additional improvements are seen when introducing a mix of Wi-Fi 5 and Wi-Fi 6 client devices. The improvements justify using Wi-Fi 6 APs today while waiting for the influx of Wi-Fi 6 devices that are coming tomorrow.
Before Wi-Fi 6, capacity constraints were dictated by the overall efficiency of the resources the devices connected to. Today, the always-on demand for wireless connectivity and mobility makes it critical to have additional capacity and efficiency—which is exactly what Wi-Fi 6 provides.
Wireless networks are no longer focused on mobile devices. An International Data Corp. study estimates that, by 2025, the world will have 41.6 billion connected IoT devices, including machines, sensors and cameras. Few of these are considered mobile. In the average enterprise, more than 30 percent of all network-connected endpoints are IoT devices (excluding mobile ones). These devices will generate nearly 80 zettabytes of data in the year 2025. For reference, one zettabyte is 1,000 x 10 kilobytes.
Additional clients with OFDMA
Instead of boosting speed for individual devices, Wi-Fi 6 is all about improving the network when multiple devices are connected. Client devices will have more opportunities to transmit and receive data—reducing latency and jitter.
In previous Wi-Fi standards, additional speed came in the form of extra transmit radios (TX), receive radios (RX) and spatial streams (SS), read as “TX x RX: SS” (4x4:4 would mean four transmitting radios, four receiving radios through four spatial streams), additional channel widths (ranging from 20 MHz to 320 MHz), or increased QAM (from 16-QAM to 1024-QAM). This extra hardware and improved modulation aren’t the only aspect that helps Wi-Fi 6 increase network speed. And the clients don’t necessarily have to be Wi-Fi 6 devices. The benefits will apply to older devices as well.
About the improved modulation: Higher data rates (network speed) implies more bits per second, which calls for a more sophisticated modulation—in the case of Wi-Fi 6, a denser QAM constellation. To properly establish communication, the radios need to hit a specific dot within the QAM constellation and do it on demand. While 64-QAM enables a slower network speed than higher QAMs, it’s much easier to hit those tiny dots in the constellation, making the system more robust. If Wi-Fi 6 relied solely on 1024-QAM to achieve an increase in speed, the improvement wouldn’t be as great as might be expected.
Error vector magnitude (EVM) is an imaginary box drawn around each dot in the quadrature amplitude modulation (QAM) constellation. Equidistant from each dot, it represents the margin of error a signal has when trying to hit the target. Since perfection in wireless is difficult to obtain, the dot in the constellation doesn’t have to hit exactly in the center.
The lower the QAM (16 versus 64), the bigger the target (the EVM), but with a decrease in value or speed. The higher the QAM (64 vs 1024), the smaller the target. Given the size of the EVM at 64 QAM, imagine the size of the EVM at 1024 QAM.
At higher QAM rates, devices need to have very “clean air” (high signal-to-noise ratio) to hit EVM every time. If the air isn’t “clean,” some devices will downshift from 1024-QAM to 256-QAM, then to 64-QAM and, finally, 16-QAM—exchanging speed for reliability. As the QAM number decreases, the EVM in the constellation gets bigger, making it an easier target to hit.
Even with older clients (non-Wi-Fi 6), a new AP will be much better at hitting its target, or EVM, in the QAM constellation, resulting in a faster experience for all devices—not just those capable of Wi-Fi 6 and 1024-QAM. With Wi-Fi 6, not all devices will be able to take advantage of 1024-QAM, but some will. Wi-Fi 6 clients that send their data faster—even some of the time—make a more efficient basic service set (Wi-Fi set of cells), which makes the experience faster for all.
By not relying solely on faster and higher QAM rates, the additional speed in Wi-Fi 6 comes with increased efficiency and capacity. As devices can use the spectrum more efficiently, it opens up time slots for devices to transmit, known as transmit opportunity (TXOP). By using OFDMA, devices with smaller payloads can transmit their data simultaneously. More opportunity on the channel means all devices will naturally speed up as more time is available for devices that need it.
Resource utilization improvement
More TXOP, combined with devices that can use higher QAM rates, means devices get more data transmitted faster—resulting in a decrease of the TXOPs the device needs. More TXOP for other devices, including Wi-Fi 4 and 5 devices, means they will go faster.
The bottom line? Upgrading to a Wi-Fi 6 network, even if the majority of clients are not Wi-Fi 6 capable, is anticipated to solve many of today’s network challenges.
Mandatory WPA3
Wi-Fi’s biggest security update, Wi-Fi Protected Access 3 (WPA3), is a suite of protocols and technologies that provide authentication and encryption for Wi-Fi networks. WPA3 makes it harder for hackers to crack passwords by guessing them. Current devices can support WPA3, but it’s optional. WPA3 is required for Wi-Fi 6 devices to receive Wi-Fi Alliance certification12.
Simultaneous authentication of equals
The updated version of WPA2-Personal (aka PSK) is WPA3 Simultaneous Authentication of Equals (SAE). SAE provides a more secure, password-based authentication and key agreement mechanism, even when passwords aren’t complex.
SAE dictates how a new device, or user, should “greet” a network access point when they exchange cryptographic keys.13 This will delay the effect of a possible attack and make the password harder to crack. The SAE also averts the possible decryption of data when offline.
Protected management frames
WPA3 requires protected management frames (PMFs) to be enabled. PMFs enhance Wi-Fi security and network protection from malicious attacks, such as spoofing, by providing data confidentiality and replay protection of management frames.
Wi-Fi 6E opens up new airwaves for Wi-Fi signals over the 6 GHz band. This should allow faster, more reliable connections with next-generation devices. The additional band essentially quadruples the number of airwaves for APs, routers and smart devices: 14 additional 80 MHz channels and seven additional 160 MHz channels. High performance, low latency, improved throughput and faster data rates will extend into the 6 GHz band.
Less congestion
Higher speed
低遅延
More wired replacement applications
Wi-Fi 6E is designed to help alleviate the congestion, bottlenecks and constrained channel width of legacy Wi-Fi bands:
- Less congestion: Current Wi-Fi offers 28 non-overlapping 20 MHz channels; Wi-Fi 6E will offer 59 new 20 MHz channels14. The added channels will help minimize many of today’s congestion challenges and enable better support for more connected devices and device types.
- Higher speed: 1,200 MHz of contiguous spectrum enables channel bonding of 80 MHz (14 new channels), and even 160 MHz (7 new channels). This is good news for high-density venues like convention centers and auditoriums. In the home, Wi-Fi and Wi-Fi 6E will deliver speeds to complement the multi-gigabit speeds of the latest fiber and DOCSIS 3.1 networks. By combining multiple 20 MHz channels into one wider, higher-throughput 80 MHz or 160 MHz channel, existing Wi-Fi 6 clients can reach their maximum speeds without the limits of operating in smaller channel widths. Wi-Fi 6E can also support more wired replacement applications, like wireless point-to-point and indoor mesh backbone links.
- Low latency: Wi-Fi 6E will support only devices capable of OFDMA; multiuser, multiple input, multiple output (MU-MIMO); 1024-QAM; and 6 GHz. All other legacy Wi-Fi devices will be limited to the 2.4 GHz and 5 GHz bands. New APs are expected to provide backward compatibility to support Wi-Fi 6E and legacy bands.
- Wired replacement applications: Wi-Fi 6E can also support more wired replacement applications, including wireless point-to-point and indoor mesh backbone links.
Key differences between Wi-Fi 5, Wi-Fi 6 and Wi-Fi 6E:
Previous modulation schemes in Wi-Fi used orthogonal frequency division multiplexing (OFDM), which only allows one device to transmit at a time, no matter the size of the payload. With one complete frame (the name for a packet while it’s in the air) being assigned to one client device—whether it needs the full frame or not—the inefficiencies are obvious.
OFDM on a 20 MHz wide channel
Wi-Fi 6 uses a new modulation scheme known as OFDMA, which now allows up to nine devices to transmit simultaneously on a 20 MHz channel, if their payloads meet the requirements. If the payload that needs to be transmitted requires more capacity, this is adjusted and scheduled on the fly by the infrastructure to allow for the most efficient combinations of payloads to be transmitted at the same time.
OFDMA on a 20 MHz wide channel
When the two figures above are compared, you can see that what had taken three frames to transport using OFDM can now be combined into one frame. What had been a half-empty resource (Frame #1 for Client A) is now filled with the additional data for two additional clients (Frames 2 and 3 for Clients B and C). Both figures still use a 20 MHz wide channel, but in Figure 3 we see the introduction of the resource unit (RU) or that 20 MHz wide channel being broken down into nine separate frequency allocations.
What had taken eight frames in OFDM can now be accomplished in just three frames of OFDMA. Wi-Fi 6’s new efficiency means that, starting at Frame #4, by using OFDMA, clients can now send more data—or, as seen in Frame #5, additional clients can now send their data. By fully utilizing the resources available, more data can be sent in the same amount of time (eight frames for each example) and more clients have an opportunity to send their data. More data from more clients in less time results in a network that feels faster than previous generations.
Of note is that not all clients need to be using (or capable of) OFDMA. Grouping the OFDMA (Wi-Fi 6) clients into a single frame opens up the remaining frames for an OFDM (Wi-Fi 5) client to utilize the resource.
In other words, Wi-Fi 6 APs are backward compatible with older Wi-Fi devices; but, since all clients can now send their data in less time, the experience will be faster.
OFDM transitioning to OFDMA
By using a Wi-Fi 6 network and taking advantage of OFDMA, Wi-Fi 6 APs would be able to handle more devices without needing to add more APs later to carry the load as device counts continue to increase.
1024-quadrature amplitude modulation (QAM) is a highly developed modulation scheme in which data is transmitted over radio frequencies. For wireless communications, QAM is a signal in which two carriers (two sinusoidal waves)—shifted in phase by 90 degrees (a quarter out of phase)—are modulated, and the resulting output consists of amplitude and phase variations. These variations are the basis of the information we see in the client devices.
Two sinusoidal waves shifted by 90 degrees
By varying these sinusoidal waves through phase and amplitude, radio engineers can construct signals that transmit an ever-higher number of bits per hertz (information per signal). Systems designed to maximize spectral efficiency care a great deal about bits/hertz efficiency and thus are always using techniques to construct ever-denser QAM constellations to increase data rates.
Put simply, higher QAM levels increase throughput capabilities in wireless devices. By varying the amplitude of the signal and the phase, Wi-Fi radios can construct the constellation diagram below that shows the values associated with different states for a 16-QAM signal.
16-QAM constellation example
While the older Wi-Fi 5 standard is limited to 256-QAM, the new Wi-Fi 6 standard incorporates an extremely high optional modulation scheme (1024-QAM), with each symbol encoding a larger number of data bits when using a dense constellation.
In real-world terms, 1024-QAM enables a 25 percent data rate increase (throughput) in Wi-Fi 6 APs and devices. Higher throughput facilitated by 1024-QAM is critical to ensuring quality of service in high-density locations.
MIMO stands for multiple input, multiple output and relates to wireless radios using multiple antennas to transmit and receive the signal. This setup allows the APs and client devices to benefit from a variety of propagation paths, hence yielding faster speeds and longer ranges. This improvement appeared with Wi-Fi 4 and is still used by higher standards.
On top of the MIMO advantages, Wi-Fi 5 also provides a multistation (MU) wireless network throughput of at least 1 Gbps and a single station throughput of at least 500 Mbps.
However, the Wi-Fi 5 implementation of the multiuser, multiple input, multiple output (MU-MIMO), had a couple of limitations, further discussed below, that affected how it worked outside of a lab—and, in the end, resulted in almost no advantages in real-world deployment.
The first limitation was the direction of the data. It was downlink only, meaning it could only be used for sending data from the AP to the client devices. Second, instead of using radio units, it used spatial streams. This means it would only work if all client devices participating in that group were in the proper orientation to the AP—sending the data to allow separation of the spatial streams.
If clients weren’t in the green areas, as shown below, MU-MIMO wouldn’t work. In addition, as beams converged, more issues arose. The final limitation: MU-MIMO was limited to a maximum of four devices at one time.
Wi-Fi 5 MU-MIMO using spatial streams
With Wi-Fi 6 using OFDMA resource units instead of spatial streams (reference Figure 5: OFDM transitioning to OFDMA), the limitations of MU-MIMO in Wi-Fi 5 have been solved. Not only does it apply in the downlink (DL) from the AP to the client, but also to the uplink (UL) from the client to the AP.
Wi-Fi 5 used spatial streams to separate the data streams—making implementation limited and tough to orchestrate. By using the smaller channel widths of the RUs, a Wi-Fi 6 system enables the receiving station, whether the AP or client, to simply tune their receiver to the smaller slice of spectrum and ignore the data being sent to the other devices.
OFDM (Wi-Fi 5) vs OFDMA MU-MIMO (Wi-Fi 6)
Target wake time (TWT) enables devices to determine when and how frequently they will wake up to send or receive data. Essentially, this allows 802.11ax15 APs to increase device sleep time and significantly improve battery life—a feature that’s important for the internet of things.
In addition to saving power on the client side, TWT enables wireless APs and devices to negotiate and define specific times to access the medium. This helps optimize spectral efficiency by reducing contention and overlap between users. The TWT mechanism first appeared in the IEEE 802.11ah “Wi-Fi HaLow” standard.
Published in 2017, the low-power standard is specifically designed to support the large-scale deployment of IoT infrastructure—such as stations and sensors—that intelligently coordinate signal sharing. The TWT feature further evolved with the IEEE 802.11ax standard, as stations and sensors are now only required to wake and communicate with the specific beacon(s) transmitting instructions for the TWT broadcast sessions they belong to. This allows the wireless IEEE 802.11ax standard16 to optimize power saving for many devices, with more reliable, deterministic and LTE-like performance.
Legacy high-density Wi-Fi deployments typically saw multiple APs assigned to the same transmission channels because of limited spectrum—an inefficient paradigm that contributed to network congestion and slowdowns. Moreover, IEEE 802.11 devices weren’t able to effectively communicate and negotiate with each other to maximize channel resources.
In contrast, Wi-Fi 6 APs are designed to optimize the efficient reuse of spectrum in high-density deployments using techniques that include basic service set (BSS) coloring. This mechanism intelligently “color codes,” or marks, shared frequencies with a number that’s included within the PHY (physical layer in the OSI stack) header that is passed between the device and the network.
In real-world terms, these color codes allow APs to decide if the simultaneous use of spectrum is permissible because the channel is showing as busy and unavailable to use when the same color is detected. This helps mitigate overlapping basic service sets (OBSS). In turn, this protocol enables a network to more effectively—and concurrently—transmit data to multiple devices in congested areas.
This objective is achieved by identifying OBSS, negotiating medium contention and determining the most appropriate interference management techniques. Coloring also allows Wi-Fi 6 APs to precisely adjust Clear Channel Assessment (CCA) parameters, including energy (adaptive power) and signal detection (sensitivity thresholds) levels.
With Wi-Fi 6, multiple APs deployed in dense device environments can collectively deliver required quality of service (QoS) to more clients with more diverse usage profiles. This is made possible by a range of technologies—such as BSS coloring—which maximizes network performance by working even within heavily congested, co-channel interference environments.
Stay tuned for more Wi-Fi 6 tech innovations in the second part of this article.
As with all technology, the question is always, “What’s coming next?”—and Wi-Fi is no exception. The IEEE’s general cycle for releasing the next generation of Wi-Fi is about six years. The IEEE17 was originally projected to finalize 802.11ax (Wi-Fi 6) in 2019 (six years after 802.11ac—Wi-Fi 5 was finalized in 2013), but the final document was delayed. Undeterred, the IEEE had already started working on the next generation of the Wi-Fi PHY standard—802.11be—which the Wi-Fi Alliance is anticipated to name “Wi-Fi 7.”
The industry expects an early release of the 802.11be (Wi-Fi 7) amendment in early 202418, with a finalized amendment coming in 2025, holding to the six-year cycle. Wi-Fi 7 is expected to build upon the features that came with Wi-Fi 6 and include the spectrum in Wi-Fi 6E, allowing for a unified standard that will include the three unlicensed bands: 2.4; 5; and 6 GHz. Wi-Fi 7 will also be known as “Extremely High Throughput,” and the reasoning will become evident soon.
While the modulation scheme won’t change like we saw from Wi-Fi 5 to Wi-Fi 6, additional features19 are expected to make it to the final amendment:
- 320 MHz-wide channel
- 4096-QAM (4K-QAM)
- 16 spatial streams
- Multi-band/multi-channel aggregation
- Enhanced resource allocation
- Optimized channel sounding to improve airtime utilization
- Multi-access point (AP) coordination (multiple APs sending to a single client)
- Contiguous and noncontiguous 320/160+160 MHz and 240/160+80 MHz bandwidth
While these aren’t guaranteed to make the final draft—and others could be added later—some of these features explain the “Extremely High Throughput” designation that Wi-Fi 7 is getting. Consider using 4096-QAM on a channel width of 320 MHz, and speeds are expected to cross the 45 Gbps range—about four times the speed of Wi-Fi 6.
320 MHz-wide channel
1024-QAM vs 4096-QAM
Along with 16 spatial streams, the ability to aggregate channels across multiple bands is also a promise for speed enhancements. Utilization improvements are also expected to enhance the efficiencies introduced in Wi-Fi 6. These improvements are mainly in the background process inherent in the CSMA-CA process used in Wi-Fi. End users will notice any improvements in efficiency as the service being “faster,” since less time is wasted not sending or receiving data.
16 spatial streams
Channel aggregation across multiple bands
Other improvements that stand out are the multi-AP coordination and the introduction of noncontiguous bandwidth features. While very promising, these could be challenging in real-world scenarios.
Multi-AP is the concept that two different APs will be able to send data to the same client at the same time—halving the time needed to send data. If it takes 90 seconds to download a movie today, that same movie could be downloaded in just 45 seconds with this one new feature. Factoring in the new 4K-QAM and a wider channel, that same movie could realistically be downloaded in less than 15 seconds.
Multi-access point coordination
Contiguous and noncontiguous 160 MHz bandwidth
The final significant feature announcement is the noncontiguous bandwidth requirement. To form a channel that’s 160 MHz wide today, the entire spectrum—from channel 36 (5.170 GHz) to channel 64 (5.330 GHz)—needs to be available without interruptions. That contiguous spectrum is required to make a channel that’s 160 MHz wide. With this new feature, 80 MHz of spectrum from the U-NII-2a band (channels 52 through 64) could be combined with the U-NII-3 band (channels 149 through 161) to form a channel that uses 160 MHz worth of spectrum even though they aren’t contiguous. Being able to use the spectrum in this way allows designers to use wider channels that aren’t in use today, so every user would have a similar experience.
Airtime and spectrum improvements enhance user experience, which is what everyone is working toward.
As with previous generations of Wi-Fi, Wi-Fi 7 consumer devices are expected to be seen on the market well before the IEEE amendment is finalized. Some forecasts are calling for Wi-Fi 7 devices to start appearing as soon as early 2023, with enterprise devices starting in the fall of 2023. Combined with the delay in the automatic frequency coordination (AFC) process needed for the outdoor 6 GHz spectrum, enterprise Wi-Fi 7 devices should slot in nicely a few months after the AFC needed for full operation is online and functioning.
1 https://www.wi-fi.org/discover-wi-fi/value-of-wi-fi
2 Wi-Fi Definition (techterms.com)
3 Wi-Fi - Wikipedia
4 Hedy Lamarr, inventor of Wi-Fi, is subject of 'Bombshell' - CNET
5 Wireless Network - an overview | ScienceDirect Topics
6 Discover Wi-Fi | Wi-Fi Alliance
7 https://wlanprofessionals.com/updated-unlicensed-spectrum-charts/
8 Number of connected devices per person | Statista
9 https://www.martechadvisor.com/articles/iot/by-2030-each-person-will-own-15-connected-devices-heres-what-that-means-for-your-business-and-content/
10 https://www.sdxcentral.com/5g/definitions/will-5g-WiFi/
11 Evaluating the New 802.11ax WiFi Standard and What It Will Mean for Enterprises (commscope.com)
12 Certification | Wi-Fi Alliance
13- https://spectrum.ieee.org/everything-you-need-to-know-about-wpa3
14- https://wlanprofessionals.com/updated-unlicensed-spectrum-charts/
15 IEEE 802.11ax-2021—IEEE Standard for Information Technology—Telecommunications and Information Exchange between Systems Local and Metropolitan Area Networks—Specific Requirements Part 11: Wireless LAN Medium Access Control (MAC) and Physical Layer (PHY) Specifications Amendment 1: Enhancements for High-Efficiency WLAN
16 IEEE 802.11, The Working Group Setting the Standards for Wireless LANs
17 IEEE P802.11—TASK GROUP BE (EHT)—GROUP INFORMATION UPDATE (ieee802.org)
18- https://en.wikipedia.org/wiki/IEEE_802.11be
19 As of this writing, these features are only hypothetical, and the IEEE can drop any feature before it reaches the final document.